The sense of smell depends on sensitive proteins embedded in the nose. In two studies published recently in Science (1,2), a team led by the Baker Lab has shown that an effectively unlimited number of new protein sensors can now be generated with the help of AI. This is a major step toward more advanced molecular sensing technologies for use in healthcare, pollution monitoring, and more.
Biochemically speaking, smelling a rose requires two feats. First, floral compounds must bind to olfactory receptors in the nose. This molecular handshake must then produce a reliable signal, leading to the perception of smell.
In the new studies, the team used computers to create custom proteins that bind to specific compounds and transmit stable bioelectronic binding signals across lipid membranes. These sensor proteins are not derived from any found in nature. Instead, they were built from scratch and confirmed to function in the lab.
“When we started with this idea a few years ago, many people thought it was impossible,” said senior author and former Baker Lab postdoc Anastassia Vorobieva, now a group leader at the VIB-VUB Center for Structural Biology in Belgium. “Now we have shown that we can successfully design nanopore proteins with a high success rate and that they can have stable and reproducible conductance.”
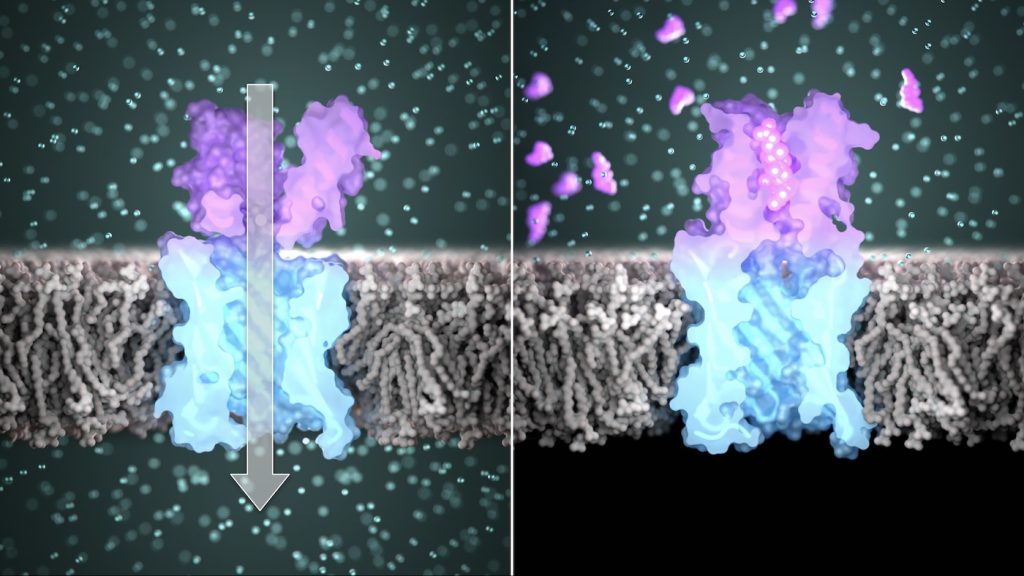
This research was led by Baker Lab researchers Samuel Berhanu, Sagardip Majumder, and Linna An. Scientists from the University of Basel, University of Leeds, University of Virginia, Lawrence Berkeley National Laboratory, VIB-VUB, and the Institute for Protein Design Core R&D Labs also contributed to the structural and functional analyses of the new proteins.
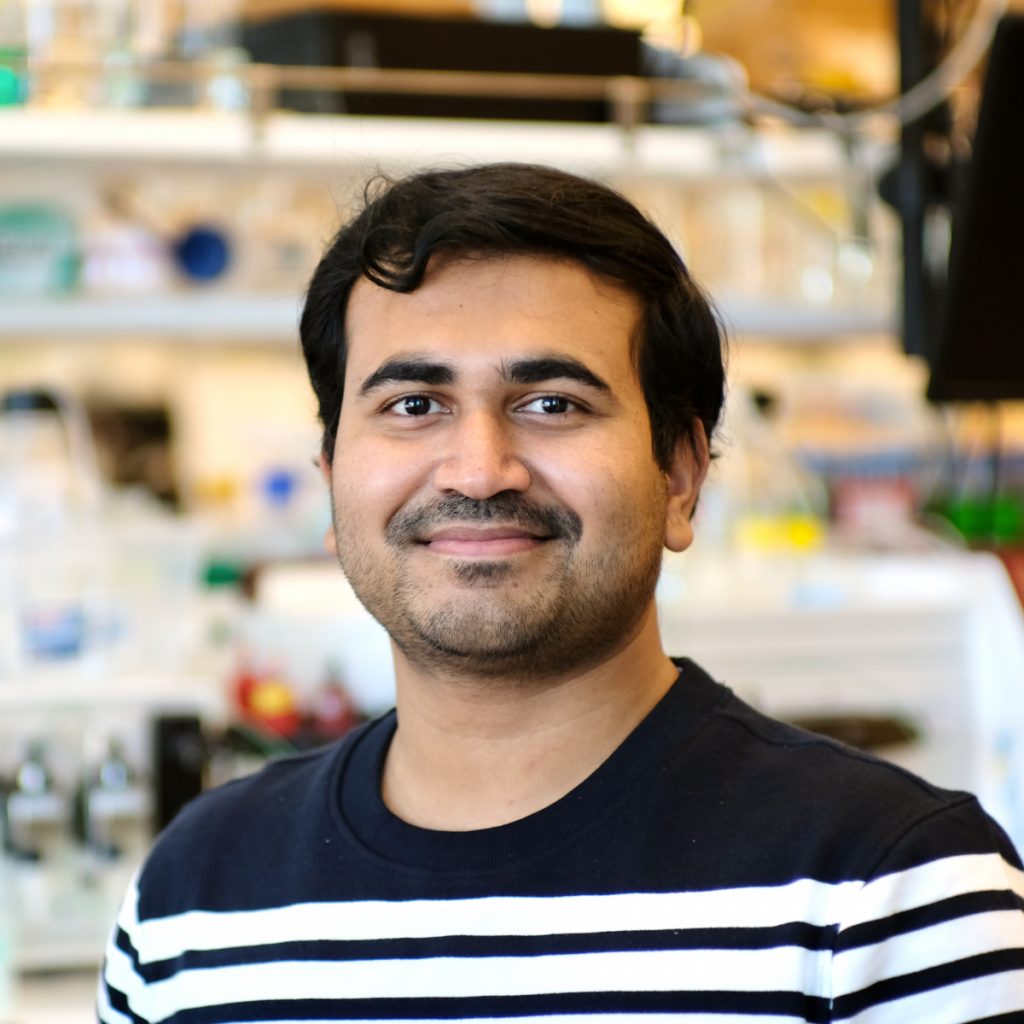
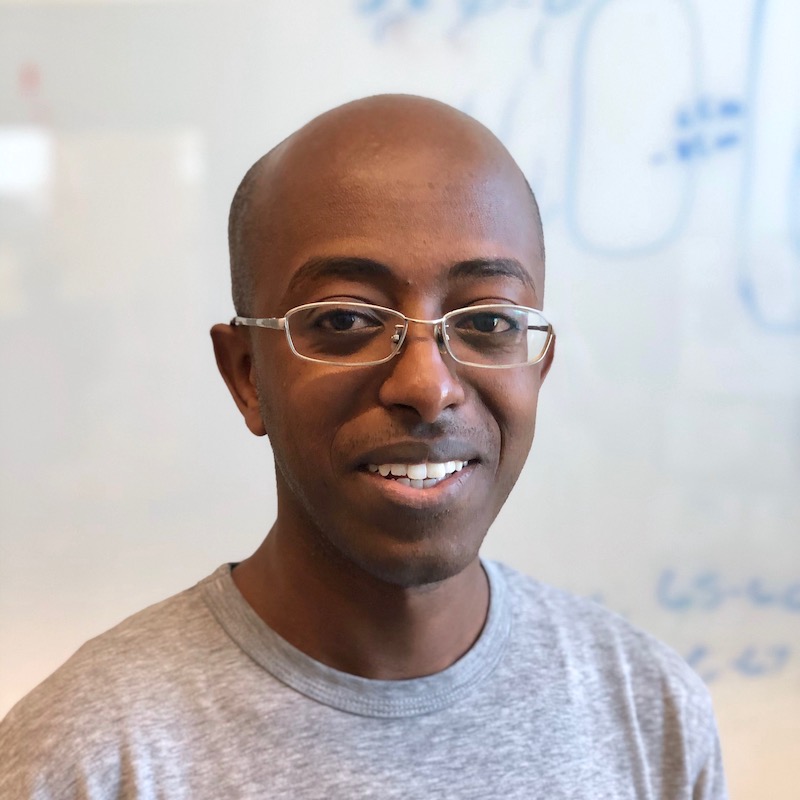
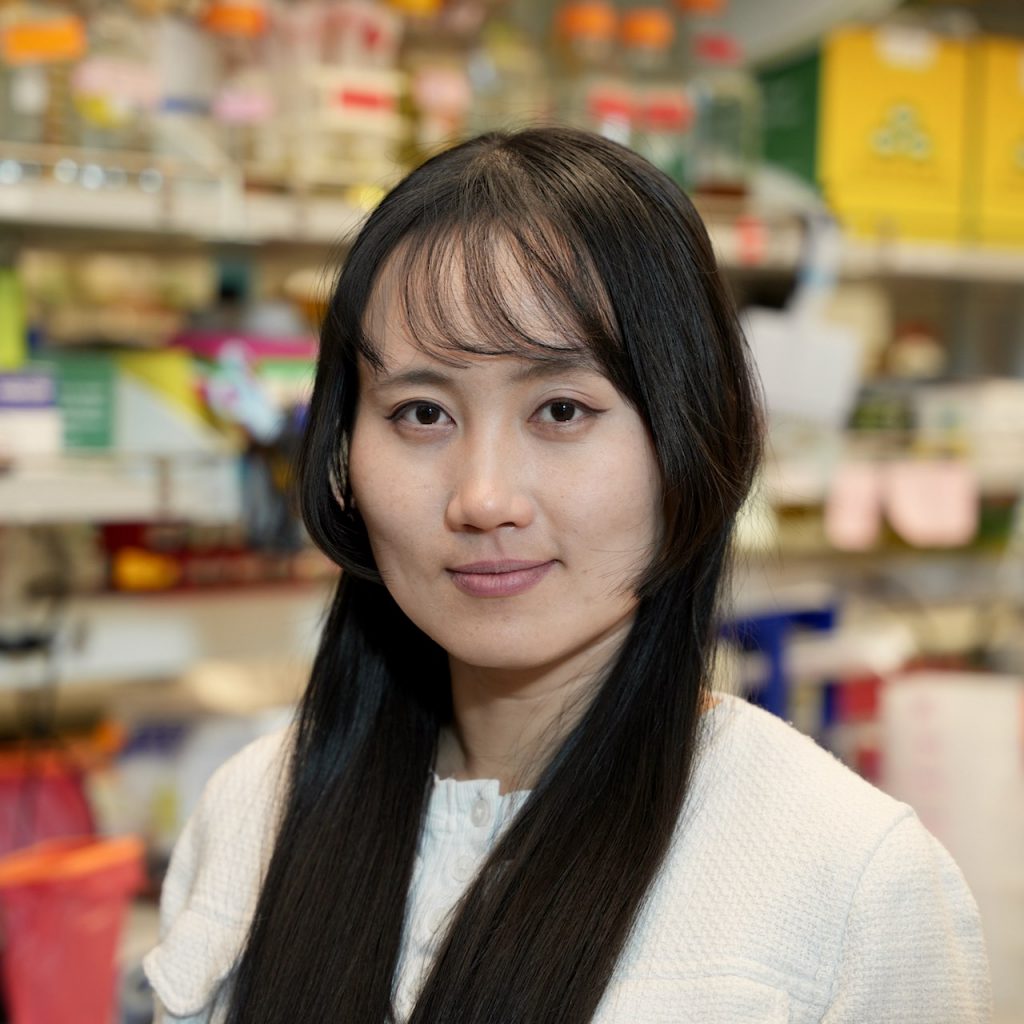
The biosensors were created in stages and reported across two papers. Together, these projects yielded entirely synthetic receptors that resemble those in the nose. “This collaboration is a great example of what’s possible with protein design,” said senior author and IPD director David Baker. “Rather than repurposing biomolecules from nature, we can now create the functions we want from first principles.”
Sculpting new nanopores
In the first study, the researchers designed custom protein nanopores and characterized them in the lab. These tube-like molecules have openings, or pores, less than three nanometers wide — roughly 20,000 times smaller than the diameter of a human hair.
The team showed that these custom nanopores embed into lipid membranes, similar to nasal scent receptors, and function as conduits for electrically charged ions as intended.
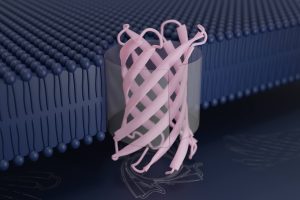
“Natural protein nanopores are important tools for DNA sequencing, but only a handful of these delicate molecules are reliable enough to work with. For this project, we created more stable nanopores than anyone’s had access to, and there’s effectively no limit to the number we could make using this design approach,” said Samuel, now a research scientist at IEH Laboratories and Consulting Group.
“This work brings biology and electronics much closer together. We’re now exploring how to incorporate them into bio-electronic devices that can detect tiny traces of chemicals, diagnose diseases, and possibly form critical components of nanoscale filtration devices,” said Sagardip.
Binding to chemicals
The second study introduced a novel approach for creating proteins that bind to specific chemicals.
The team chose four challenging small molecules as binding targets. These included cholic acid, an important marker for liver disease; methotrexate, an anti-folate cancer treatment that requires regular blood monitoring; and thyroxine, a hormone used to diagnose thyroid conditions.
“It hasn’t been easy to detect chemicals like these, but we succeeded in generating proteins that recognize each of our four targets,” said Linna. “The same approach could be used to create sensors for virtually any small molecule.”
Using a variety of deep-learning tools, the team generated “pseudocycle” proteins. These consist of repeating structural units that surround central binding pockets of varying shapes. They then docked target chemicals into these pockets and optimized the interaction surfaces using LigandMPNN, a deep-learning tool expanded from ProteinMPNN. The highest affinity binding proteins were identified via laboratory screening.
Creating synthetic sensors
By combining the small molecule binders within the new nanopores, the team created proteins that change conductance in response to target molecules — essentially creating synthetic tools that act like olfactory receptors.
Linna believes that synthetic nanopore sensors are just one technology that will emerge from this research. “When I talk to other scientists, they’re excited by how these binding proteins may be used in many different detection systems,” she commented. In addition to the nanopore sensors, the team describes chemically induced dimerization (CID) systems that could be used to control cancer cell therapies and more.
This research opens the door to developing highly specific biosensors for detecting disease biomarkers, monitoring environmental pollutants, and advancing personal healthcare devices. The collaboration underscores the interdisciplinary nature of modern science and the potential for computational protein design to revolutionize how we create solutions to important challenges in medicine and beyond.
This research was supported by several federal, private, and philanthropic organizations. All funding sources are listed in the manuscripts.
Berhanu S, Majumder S, Müntener T, et al. Sculpting conducting nanopore size and shape through de novo protein design. Science, 18 July 2024
Ah L, et al. Binding and sensing diverse small molecules using shape-complementary pseudocycles. Science, 18 July 2024